Addressing Geotechnical Challenges in Bridge Foundation Design and Rehabilitation
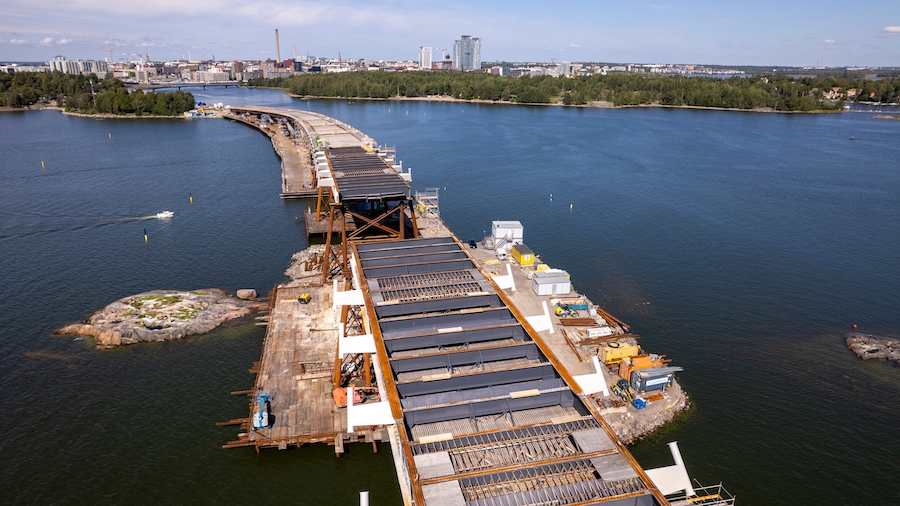
View the complete article here.
The significance of bridge foundation design and refurbishment cannot be overstated—considering their impact on the safety, endurance, and operational efficacy of indispensable transportation networks. In this guide, we will address the challenges posed by varying soil conditions, the importance of geotechnical investigations, and the rehabilitation imperative for aging infrastructure. Furthermore, we will discuss the techniques of soil stabilization, ground improvement, and the critical role played by deep foundations in bridge construction.
Bridge Foundation Types and Their Importance
Bridge foundations are the fundamental building blocks upon which the stability and structural integrity of bridges depend. These foundations are tailored to accommodate specific site conditions, load-bearing requirements, and environmental considerations. Understanding the wide array of bridge foundation types is essential—as selecting the appropriate type is a critical factor in ensuring a bridge’s safety, resilience, and longevity.
Types of Bridge Foundations
- Shallow foundations (spread footings):
- Strip footings: Strip footings distribute the bridge’s load across a wider area when the upper layer of soil is robust enough to support the loads. They are commonly used in various shapes and configurations.
- Mat foundations (raft foundations): Mat foundations—also known as raft foundations—are large, solid slabs that evenly distribute the bridge loads. They are particularly suitable for areas with weak or compressible soils.
- Slab-on-grade foundations: Slab-on-grade foundations involve a single, continuous concrete slab that supports the bridge superstructure. They are frequently used for low-profile bridges.
- Trench foundations: Trench foundations are long, narrow footings used to support the bridge structure. They are suitable for bridges with specific design requirements.
- Deep foundations:
- Piles (driven piles, drilled shafts, helical piles, etc.): Piles are slender structural elements made of materials such as concrete, steel, or timber. They extend deep into the ground to transfer the bridge’s load to more stable soil or bedrock layers. Various pile types—including driven piles, drilled shafts, and helical piles—are employed depending on the project’s needs.
- Caissons (drilled caissons, floating caissons, etc.): Caissons are large cylindrical concrete structures that are either drilled into the ground or used as floating foundations. They are employed in deep-water or high-load situations, such as bridge piers over rivers or bays.
- Piers (pier shafts, pier columns): Piers are vertical columns or shafts that provide support for bridges with multiple piers. They can be shallow or deep foundations, depending on factors like pier height and soil conditions.
- Micropiles: Micropiles are small-diameter piles typically used in limited access or environmentally sensitive areas. They are installed using drilling techniques and are suitable for retrofitting existing structures.
- Tension piles: Tension piles are utilized to resist uplift forces in bridges, especially in areas prone to seismic activity or high winds.
- Abutment foundations:
- Pier foundations: Pier foundations are located at the ends of bridges and support the bridge’s superstructure. They are typically shallow foundations.
- Floating foundations: Floating foundations are employed for bridges constructed over bodies of water with soft or unstable sediment at the bottom. They allow the bridge to “float” on the water’s surface, providing stability without reaching bedrock.
- Mat foundations (raft foundations): Mat foundations are large slabs that distribute the bridge loads over a broad area and are also used in abutment foundations.
- Group piles: Group piles consist of clusters of individual piles working together to support the bridge, often used when loads are too high for single piles.
- Well foundations (used in water crossings): Well foundations are employed in water crossings, where cylindrical structures are sunk into the waterbed and filled with concrete to provide support.
- Sheet pile foundations: Sheet pile foundations use interlocking steel or concrete sheets driven into the ground to create a retaining wall, often employed in water-related projects.
- Cofferdams (used for construction in water): Cofferdams are temporary watertight enclosures used to create dry workspaces in water for construction.
Why Choosing the Appropriate Foundation Type is Crucial for Bridge Stability
Selecting the right foundation type is of paramount importance for several reasons…
Foundation types must align with specific soil conditions at the construction site. Inadequate foundations can lead to settlement, instability, and potential bridge failure.
Different bridge designs necessitate specific foundation types to evenly distribute the loads from the superstructure to the ground. An improper choice can result in uneven stress distribution and structural issues.
Environmental factors such as water tables, seismic activity, and soil erosion significantly impact foundation selection. The wrong choice can leave the bridge vulnerable to devastating environmental hazards.
Foundation types directly influence a bridge’s durability and longevity. The right foundations can ensure the bridge’s stability and functionality for decades or even centuries.
Geotechnical Challenges in New Bridge Construction
New bridge construction projects can bring a host of geotechnical challenges, largely stemming from the complexities of soil conditions and the site-specific nature of each endeavor.
Complexities of Soil Conditions and Site-Specific Challenges
The soil beneath a bridge site is the very foundation upon which the entire structure will rest. However, soil conditions can vary significantly from one location to another—presenting unique challenges that demand careful consideration…
Soil properties can change dramatically over relatively short distances. Engineers must contend with variations in soil type, composition, bearing capacity, and settlement potential.
Some bridge sites may feature weak or compressible soils that lack the necessary load-bearing capacity to support the structure. This may require the implementation of deep foundations or ground improvement techniques.
High groundwater tables or the potential for artesian conditions can complicate foundation design and construction, potentially leading to buoyancy concerns or soil liquefaction during seismic events.
In regions prone to seismic activity, the soil’s response to ground motion becomes a critical factor. Engineers must account for soil liquefaction potential, site-specific amplification effects, and foundation settlement during an earthquake.
Site-specific environmental factors—such as the presence of wetlands, protected habitats, or archaeological sites—may necessitate specialized foundation design and construction techniques to minimize ecological impact.
Importance of Site Investigations and Geotechnical Surveys
In the face of these geotechnical challenges, comprehensive site investigations and geotechnical surveys are indispensable. These activities provide critical insights into the subsurface conditions and lay the groundwork for informed decision-making.
Through soil borings, test pits, and geophysical surveys—engineers can characterize the soil and rock properties at the site. This information helps in assessing bearing capacity, settlement potential, and seismic vulnerability.
Geotechnical investigations enable engineers to tailor the bridge’s foundation design to the specific soil conditions, ensuring that it can safely distribute the loads and resist settlement.
Geotechnical surveys also assist in identifying potential hazards, such as landslides or soil liquefaction, allowing engineers to develop risk mitigation strategies.
Accurate knowledge of the subsurface conditions is vital for construction planning. It helps in selecting the appropriate construction methods, equipment, and materials.
Geotechnical data informs cost estimates, allowing project planners to allocate resources effectively and avoid unexpected expenses related to foundation issues.
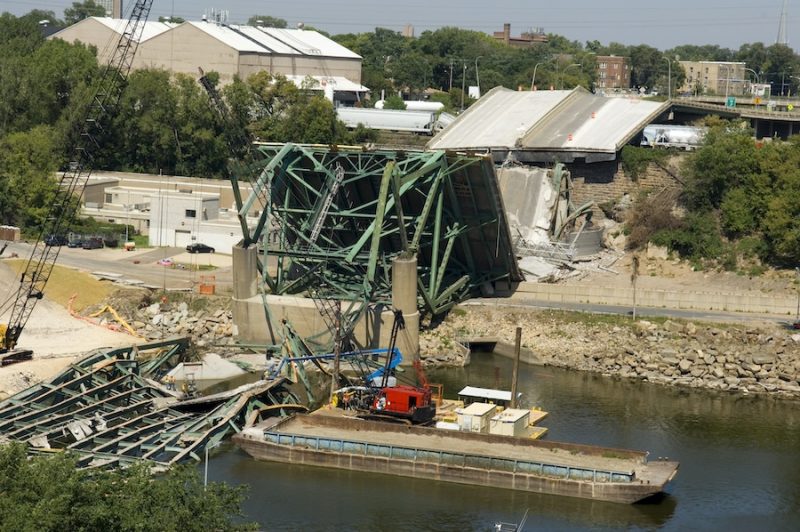
Rehabilitation of Existing Bridge Foundations
The rehabilitation of existing bridge foundations is a critical aspect of maintaining and extending the service life of aging infrastructure.
Highlighting the Need for Rehabilitation Due to Aging Infrastructure
As our nation’s bridge infrastructure continues to age, the need for rehabilitation becomes increasingly evident. Many existing bridges were built decades ago and are now facing a myriad of issues related to their foundations…
Over time, bridge foundations can deteriorate due to various factors—including exposure to environmental conditions, increased traffic loads, and natural wear-and-tear. This deterioration can compromise the integrity and safety of the entire bridge structure.
As traffic loads and transportation demands evolve, older bridge foundations may not meet current load-bearing requirements. Rehabilitation is often necessary to bring these structures up to modern standards.
The materials used in older bridge foundations may no longer meet current engineering standards—leading to issues such as corrosion, rust, or decay. Rehabilitation efforts can combat this material degradation.
Older bridges were often designed based on outdated standards and codes. Rehabilitating the foundations allows for the incorporation of modern design principles, improving overall safety and performance.
Common Problems and Deterioration Factors in Bridge Foundations
The rehabilitation of bridge foundations typically becomes necessary due to a range of common problems and deterioration factors…
The exposure to moisture and de-icing salts can lead to corrosion of steel and other materials used in the foundation. This can weaken the foundation’s structural integrity and reduce its load-bearing capacity.
Over time, foundations may experience uneven settlement—leading to misalignment and reduced stability. Differential settlement can cause structural distress and pose safety risks.
Cracks in foundation elements, caused by factors like freeze-thaw cycles or soil erosion, can compromise their strength and durability.
Older bridges may not have been designed to withstand seismic events. Rehabilitation can include seismic retrofitting to enhance their resilience.
For bridges located in areas prone to slope erosion, the foundation’s stability can be threatened. Measures such as slope stabilization may be required as part of rehabilitation efforts.
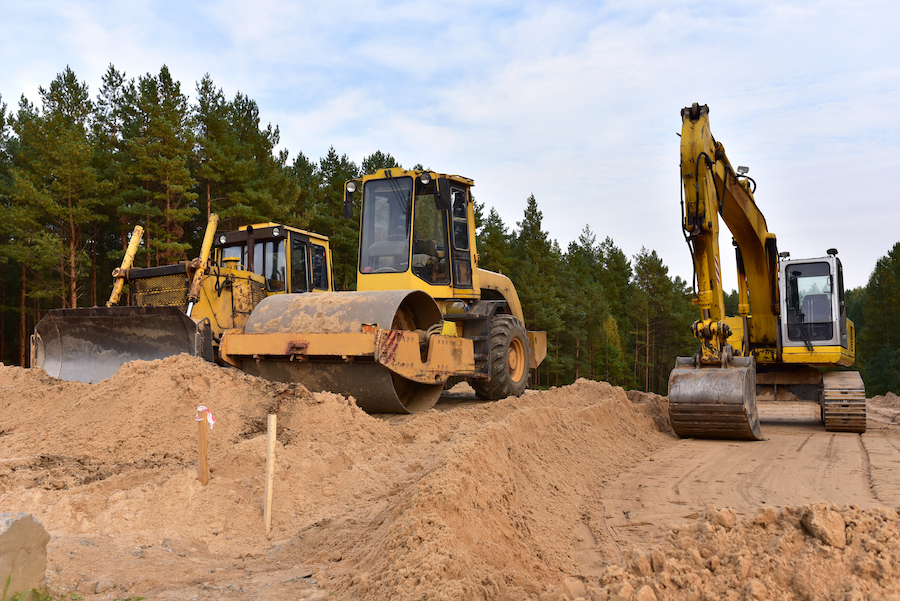
Soil Stabilization Techniques
Soil stabilization is the process of altering soil properties to improve its engineering characteristics. This is essential for creating a stable foundation for bridge structures. Here are some soil stabilization methods commonly employed in bridge foundation design and rehabilitation…
Compaction
Compaction involves mechanically densifying soil by applying force or vibration to reduce its void ratio. This increases the soil’s shear strength and bearing capacity. Compaction is frequently used to prepare foundation beds or to enhance the load-bearing capacity of existing foundations.
Grouting
Grouting is the injection of a cementitious or chemical mixture into the soil to fill voids and fractures. It improves soil cohesion, increases bearing capacity, and stabilizes foundations. Grouting is used for various purposes, including underpinning existing foundations and sealing groundwater pathways.
Chemical Stabilization
Chemical stabilization techniques involve the addition of chemical agents, such as lime or cement, to the soil to alter its properties. These chemicals react with the soil particles—improving its strength, plasticity, and durability. Chemical stabilization is particularly useful for weak or expansive soils.
Reinforcement
Soil reinforcement techniques involve the introduction of geosynthetic materials like geotextiles, geogrids, or soil nails into the soil. These materials enhance the soil’s tensile strength and load-bearing capacity. Soil reinforcement is often used in slope stabilization and retaining wall construction.
Deep Foundation Solutions
Deep foundation solutions, including piles and drilled shafts, are integral components of many bridge construction projects.
The Role of Deep Foundations in Bridge Projects
Deep foundations serve as the cornerstone of bridge projects, providing the necessary support and stability when shallow foundations are insufficient. Deep foundations are essential for the following reasons…
In areas where the upper layer of soil lacks the necessary bearing capacity, deep foundations are used to transfer the bridge’s load to deeper, more stable soil or bedrock layers.
Deep foundations help distribute the bridge’s load over a larger area, reducing the risk of differential settlement, which can lead to structural distortion and damage.
In locations with high groundwater tables, deep foundations are crucial for preventing buoyancy issues that could threaten the bridge’s stability.
Deep foundations are versatile and can be designed to minimize environmental impacts, making them suitable for use in sensitive ecological areas or water crossings.
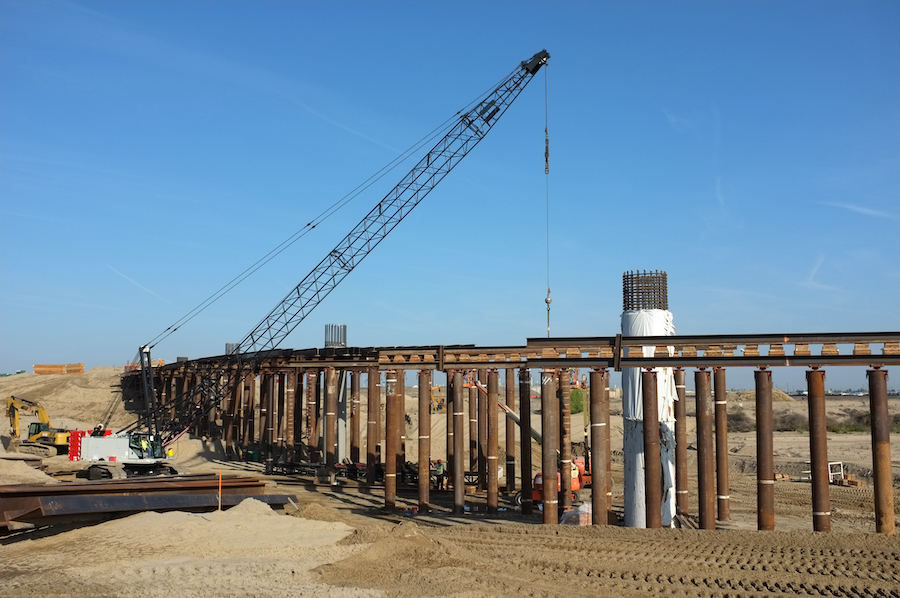
Design Considerations and Load-Bearing Capacity
Designing deep foundations for bridge projects involves a series of critical considerations, with load-bearing capacity being a primary focus.
Engineers perform geotechnical investigations to assess soil conditions and determine the depth at which stable soil or bedrock can be reached. This analysis informs foundation design.
Based on the geotechnical analysis, the appropriate deep foundation type is selected. Factors such as soil composition, groundwater levels, and expected loads play a crucial role in this decision.
The design must ensure that the load from the bridge superstructure is evenly distributed to the deep foundation elements. Proper load distribution prevents overstressing and settlement issues.
Deep foundations are constructed using various materials—including concrete, steel, or timber. The choice of material depends on factors like load requirements, environmental conditions, and project budget.
Construction methods for deep foundations vary based on the selected foundation type. For example, driven piles are mechanically driven into the ground—while drilled shafts are created by excavating and filling with concrete.
Load testing is often conducted to validate the load-bearing capacity of the deep foundation elements. This ensures that the foundations can safely support the anticipated bridge loads.
Continuous monitoring and periodic maintenance are essential to assess the performance of deep foundations over time and address any issues that may arise.
Conclusion
Bridge foundation design and rehabilitation both demand careful consideration of a multitude of factors. The selection of the appropriate foundation type is pivotal, as it forms the bedrock upon which the entire bridge structure rests—impacting its safety, longevity, and resilience. Soil stabilization techniques and ground improvement methods offer essential tools for enhancing the performance of bridge foundations, allowing them to adapt to various geotechnical challenges. Furthermore, deep foundation solutions—including piles and drilled shafts—provide essential support and stability, with meticulous design considerations ensuring their ability to bear the required loads. In the dynamic field of geotechnical engineering, understanding and mastering these foundational aspects is fundamental to the continued growth and maintenance of our bridges and other types of infrastructure.
View the complete article here.
What are the common problems and deterioration factors that may lead to the rehabilitation of bridge foundations?
Common problems include corrosion, uneven settlement, cracks, outdated design standards, and susceptibility to seismic events.
How do geotechnical investigations contribute to effective bridge foundation design?
Geotechnical investigations provide crucial insights into soil conditions, aiding in foundation design by assessing bearing capacity, settlement potential, and identifying potential hazards.