Chapter 4 – Disposal Alternatives
For a complete version of this document click here.
4-1. Introduction
a. While selection of proper dredging equipment and techniques is essential for economic dredging, the selection of a disposal alternative is of equal or greater importance in determining viability of the project, especially from the environmental standpoint. There are three major disposal alternatives available:
(1) Open-water disposal.
(2) Confined disposal.
(3) Habitat development.
Each of the major disposal alternatives involves its own set of unique considerations, and selection of a disposal alternative should be made based on both economic and environmental considerations.
b. This chapter describes considerations in evaluation of disposal alternatives, primarily from an environmental standpoint. Sections on evaluation of pollution potential and sediment resuspension due to dredging apply to all disposal alternatives, while separate sections describe considerations of each of the three major disposal alternatives.
Section I. Evaluation of Dredged Material Pollution Potential
4-2. Influence of Disposal Conditions on Environmental Impact
a. As discussed in WES TR DS-78-6, the properties of a dredged sediment affect the fate of contaminants, and the short- and long-term physical and chemical environment of the dredged material at the disposal site influences the environmental consequences of contaminants. These factors should be considered in evaluating the environmental risk of a proposed disposal method for contaminated sediment. The processes involved with release or immobilization of most sediment-associated contaminants are regulated to a large extent by the physical-chemical environment and the related bacteriological activity associated with the dredged material at the disposal site. Important physical-chemical parameters include pH, oxidation-reduction conditions, and salinity. Where the physical-chemical environment of a contaminated sediment is altered by disposal, chemical and biological processes important in determining environmental consequences of potentially toxic materials may be affected.
b. The major sediment properties that will influence the reaction of dredged material with contaminants are the amount and type of clay; organic matter content; amount and type of cations and anions associated with the sediment; the amount of potentially reactive iron and manganese; and the oxidation-reduction, pH, and salinity conditions of the sediment. Although each of these sediment properties is important, much concerning the release of contaminants from sediments can be inferred from the clay and organic matter content, initial and final pH, and oxidation-reduction conditions. Much of the dredged material removed during harbor and channel maintenance dredging is high in organic matter and clay and is both biologically and chemically active. It is usually devoid of oxygen and may contain appreciable sulfide. These sediment conditions favor effective retention of many contaminants, provided the dredged materials are not subject to mixing, resuspension, and transport. Sandy sediments low in organic matter content are much less effective in retaining metal and organic contaminants. These materials tend not to accumulate contaminants unless a contamination source is nearby. Should contamination of these sediments occur, potentially toxic substances may be readily released upon mixing in a water column, or by leaching and possibly plant uptake under intertidal or upland disposal conditions.
c. Many contaminated sediments are reducing and near neutral in pH, initially. Disposal into quiescent waters will generally maintain these conditions and favor contaminant retention. Certain sediments (noncalcareous and containing appreciable reactive iron and particularly reduced sulfur compounds) may become moderately to strongly acid upon gradual drainage and subsequent oxidation as may occur under upland disposal conditions. This altered disposal environment greatly increases the potential for releasing potentially toxic metals. In addition to the effects of pH changes, the release of most potentially toxic metals is influenced to some extent by oxidation reduction conditions, and certain of the metals can be strongly affected by oxidation-reduction conditions. Thus, contaminated sandy, low organic-matter-content sediments pose the greatest potential for release of contaminants under all conditions of disposal. Sediments which tend to become strongly acid upon drainage and long-term oxidation also pose a high environmental risk under some disposal conditions. The implications of the influence of disposal conditions on contaminant mobility are discussed below.
4-3. Methods of Characterizing Pollution Potential
a. Bioassay. Bioassay tests are used to determine the effects of a contaminant(s) on biological organisms of concern. They involve exposure of the test organisms to dredged material (or some fraction such as the elutriate) for a specified period of time, followed by determination of the response of the organisms. The most common response of interest is death. Often the tissues of organisms exposed to dredged material are analyzed chemically to determine whether they have incorporated, or bioaccumulated, any contaminants from the dredged material. Bioassays provide a direct indication of the overall biological effects of dredged material They reflect the cumulative influence of all contaminants present, including any possible interactions of contaminants. Thus, they provide an integrated measurement of potential biological effects of a dredged material discharge. For precisely these reasons, however, a bioassay cannot be used to identify the causative agent(s) of impact in a dredged material. This is of interest, but is seldom of importance, since usually the dredged material cannot be treated to remove the adverse components even if they could be identified. Dredged material bioassay techniques for aquatic animals have been implemented in the ocean-dumping regulatory program for several years (item 1) and are easily adapted for use in fresh water. Dredged material bioassays for wetland and terrestrial plants have also been developed (item 2) and are coming into ever-wider use.
b. Water Column Chemistry. Chemical constituents contained in or associated with sediments are unequally distributed among different chemical forms depending on the physicalchemical conditions in the sediments and the overlying water. When contaminants introduced into the water column become fixed into the underlying sediments, they rarely if ever become part of the geological mineral structure of the sediment. Instead, these contaminants remain dissolved in the sediment interstitial water, or pore water, become absorbed or adsorbed to the sediment ion exchange portion as ionized constituents, form organic complexes, and/or become involved in complex sediment oxidation-reduction reactions and precipitations. The fraction of a chemical constituent that is potentially available for release to the water column when sediments are disturbed is approximated by the interstitial water concentrations and the loosely bound (easily exchangeable) fraction in the sediment. The elutriate test is a simplified simulation of the dredging and disposal process wherein predetermined amounts of dredging site water and sediment are mixed together to approximate a dredged material slurry. The elutriate is analyzed for major dissolved chemical constituents deemed critical for the proposed dredging and disposal site after taking into account known sources of discharges in the area and known characteristics of the dredging and disposal site. Results of the analysis of the elutriate approximate the dissolved constituent concentration for a proposed dredged material disposal operation at the moment of discharge. These concentrations can be compared to water quality standards and mixing zone considerations to evaluate the potential environmental impact of the proposed discharge activity in the discharge area.
c. Total or Bulk Sediment Chemistry. The results of these analyses provide some indication of the general chemical similarity between the sediments to be dredged and the sediments at the proposed disposal site. The total composition of sediments, when compared with natural background levels at the site, will also, to some extent, reflect the inputs to the waterway from which they were taken and may sometimes be used to identify and locate point source discharges. Since chemical constituents are partitioned among various sediment fractions, each with its own mobility and biological availability, a total sediment analysis is not a useful index of the degree to which dredged material disposal will affect water quality or aquatic organisms. Total sediment analysis results are further limited because they cannot be compared to any established water quality criteria in order to assess the potential environmental impact of discharge operations. This is because the water quality criteria are based on water-soluble chemical species, while chemical constituents associated with dredged material suspensions are generally in particulate/solid-phase forms or mineralogical forms that have markedly lower toxicities, mobilities, and chemical reactivities than the solution-phase constituents. Consequently, little information about the biological effects of solid-phase and mineral constituents that make up the largest fraction of dredged material can be gained from total or bulk sediment analysis.
4-4. Factors Influencing Dredging Turbidity
a. Occurrence and Extent. The nature, degree, and extent of sediment suspension around a dredging or disposal operation are controlled by many factors, as discussed in WES TR DS-78-13. Chief among these are: the particle size distribution, solids concentration, and composition of the dredged material; the dredge type and size, discharge/cutter configuration, discharge rate, and solids concentration of the slurry; operational procedures used; and finally the characteristics of the hydraulic regime in the vicinity of the operation, including water composition, temperature and hydrodynamic forces (i.e., waves, currents, etc.) causing vertical and horizontal mixing. The relative importance of the different factors may vary significantly from site to site.
b. Hopper Dredge. Resuspension of fine-grained maintenance dredged material during hopper dredging operations is caused by the dragheads as they are pulled through the sediment, turbulence generated by the vessel and its prop wash, and overflow of turbid water during hopper filling operations. During the filling operation, dredged material slurry is often pumped into the hoppers after they have been filled with slurry in order to maximize the amount of solid material in the hopper. The lower density, turbid water at the surface of the filled hoppers overflows and is usually discharged through ports located near the waterline of the dredge. In the vicinity of hopper dredges during maintenance operations, a near-bottom turbidity plume of resuspended bottom material may extend 2300 to 2400 ft downcurrent from the dredge. In the immediate vicinity of the dredge, a well-defined, upper plume is generated by the overflow process. Approximately 1000 ft behind the dredge the two plumes merge into a single plume (fig. 4-1). Suspended solid concentrations above ambient may be as high as several tens of parts per thousand (grams per litre) near the discharge port and as high as a few parts per thousand near the draghead. Turbidity levels in the near-surface plume appear to decrease exponentially with increasing distance from the dredge due to settling and dispersion, quickly reaching concentrations less than 1 ppt. However, plume concentrations may exceed background levels even at distances in excess of 4000 ft.
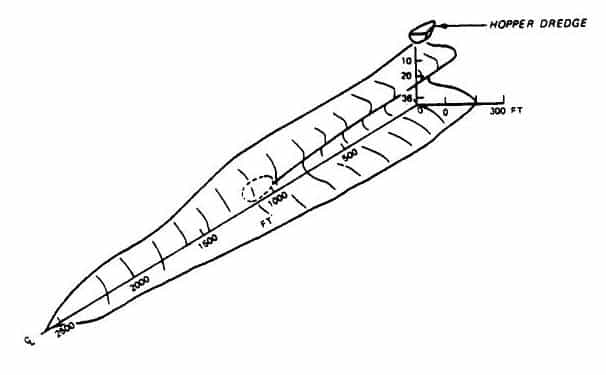
of a hopper dredge operation with overflow in
San Francisco Bay (all distances in feet).
c. Bucket or Clamshell Dredge. The turbidity generated by a typical clamshell operation can be traced to sediment resuspension occurring when the bucket impacts on and is pulled off the bottom, turbid water spills out of the bucket or leaks through openings between the jaws, and material is inadvertently spilled during the barge loading operation. There is a great deal of variability in the amount of material resuspended by clamshell dredges due to variations in bucket size, operating conditions, sediment types, and hydrodynamic conditions at the dredging site. Based on limited measurements, it appears that, depending on current velocities, the turbidity plume downstream of a typical clamshell operation may extend approximately 1000 ft at the surface and 1600 ft near the bottom. Maximum concentrations of suspended solids in the surface plume should be less than 0.5 ppt in the immediate vicinity of the operation and decrease rapidly with distance from the operation due to settling and dilution of the material. Average water-column concentrations should generally be less than 0.1 ppt. The near-bottom plume will probably have a higher solids concentration, indicating that resuspension of bottom material near the clamshell impact point is probably the primary source of turbidity in the lower water column. The visible near-surface plume will probably dissipate rapidly within an hour or two after the operation ceases.
d. Cutterhead or Hydraulic Pipeline Dredge. Most of the turbidity generated by a cutterhead dredging operation is usually found in the vicinity of the cutter. The levels of turbidity are directly related to the type and quantity of material cut, but not picked up, by the suction. The ability of the dredge’s suction to pick up bottom material determines the amount of cut material that remains on the bottom or suspended in the water column. In addition to the dredging equipment used and its mode of operation, turbidity may be caused by sloughing of material from the sides of vertical cuts; inefficient operational techniques; and the prop wash from the tenders (tugboats) used to move pipeline, anchors, etc., in the shallow water areas outside the channel. Based on limited field data collected under low current conditions, elevated levels of suspended material appear to be localized in the immediate vicinity of the cutter as the dredge swings back and forth across the dredging site. Within 10 ft of the cutter, suspended solids concentrations are highly variable but may be as high as a few tens of parts per thousand; these concentrations decrease exponentially from the cutter to the water surface. Near-bottom suspended solids concentrations may be elevated to levels of a few tenths of a part per thousand at distances of less than 1000 ft from the cutter.
Section III. Open-Water Disposal
4-5. Behavior of Discharges from Various Types of Dredges
a. Hopper Dredge. The characteristics and operation of hopper dredges are discussed in para 3-3 of this manual. When the hoppers have been filled as described, the dragarms are raised and the hopper dredge proceeds to the disposal site. At the disposal site, hopper doors in the bottom of the ship’s hull are opened and the entire hopper contents are emptied in a matter of seconds; the dredge then returns to the dredging site to reload. This procedure produces a series of discrete discharges at intervals of perhaps one to several hours. Upon release from the hopper dredge at the disposal site, the dredged material falls through the water column as a well-defined jet of high density fluid which may contain blocks of solid material. Ambient water is entrained during descent. After it hits bottom, some of the dredged material comes to rest. Some material enters the horizontally spreading bottom surge formed by the impact and is carried away from the impact point until the turbulence of the surge is sufficiently reduced to permit its deposition.
b. Bucket or Clamshell Dredge. Bucket dredges remove the sediment being dredged at nearly its in situ density and place it in barges or scows for transportation to the disposal area, as described in para 3-8. Although several barges may be used so that the dredging is essentially continuous, disposal occurs as a series of discrete discharges. The dredged material may be a slurry similar to that in a hopper dredge, but often sediments dredged by clamshell remain in fairly large consolidated clumps and reach the bottom in this form. Whatever its form, the dredged material descends rapidly through the water column to the bottom, and only a small amount of the material remains suspended.
c. Cutterhead or Hydraulic Pipeline Dredge. The operation of a cutter-head dredge, described in para 3-4, produces a slurry of sediment and water discharged at the disposal site in a continuous stream. As the dredge progresses up the channel, the pipeline is moved periodically to keep abreast of the dredge. The discharged dredged material slurry is generally dispersed in three modes. Any coarse material, such as gravel, clay balls, or coarse sand, will immediately settle to the bottom of the disposal area and usually accumulates directly beneath the discharge point. The vast majority of the fine-grained material in the slurry also descends rapidly to the bottom in a well defined jet of high-density fluid, where it forms a low-gradient circular or elliptical fluid mud mound. Approximately 1 to 3 percent of the discharged material is stripped away from the outside of the slurry jet as it descends through the water column and remains suspended as a turbidity plume.
4-6. Dredged Material Dispersion at the Discharge Site
a. Water-Column Turbidity. The levels of suspended solids in the water column around a discharge operation generally range from a few hundredths to a few tenths of a part per thousand. Concentrations are highest near the discharge point and rapidly decrease with increasing distance…
-
SPW911 Sheet Pile Design Software
Rated 5.00 out of 5$150.00 – $599.99 -
Sheet Pile Design (PDF Download)
Rated 5.00 out of 5$89.00 -
Pile Driving (PDF Download) $89.00
-
Marine Construction Volumes 1 & 2 (PDF Download) $157.00
-
Pile Driving (Book) $89.00